Femtosecond optical doping reveals new macroscopic quantum phases
Characterizing and understanding the emergence of multiple macroscopically ordered electronic phases through subtle tuning of temperature, pressure, and chemical doping has been a long-standing central issue for complex materials research. In a recent Science Advances article, we describe a high-fidelity approache that combine femtosecond electron crystallography and controlled femtosecond photo-doping to reveal the ultrafast structural dynamics of complex quantum phases in response to photonic tuning of interaction energy as an alternative route to chemical doping or applying pressure, but without uncontrolled effects due to strain or disorder. We report a comprehensive study of optical doping–induced emergence of stable phases and metastable hidden phases in charge-density wave tantalum disulfide materials. The electronic phase transitions are triggered by mid-infrared pulses, and a temperature–optical density phase diagram is constructed and substantiated with the dynamics of metastable states, highlighting the cooperation and competition through which the macroscopic quantum orders emerge. These results elucidate key pathways of femtosecond electronic switching phenomena and provide an avenue to comprehensively investigate optical doping–induced transition states. Furthermore, the speed and degree of photodoping substantially exceed that achievable with conventional methods, creating opportunities to generate new phases. The robust nonthermal switching at mesoscales and at ultrafast time scales may oneday provide a platform for designing high-speed low-energy consumption nanophotonics and electronics devices.
This work is a result of collaboration between Ruan, Duxbury, and Mahanti groups at MSU, and Kanatzidis group at Northwestern University. (Science Advances 1, e1400173 (2015)) |
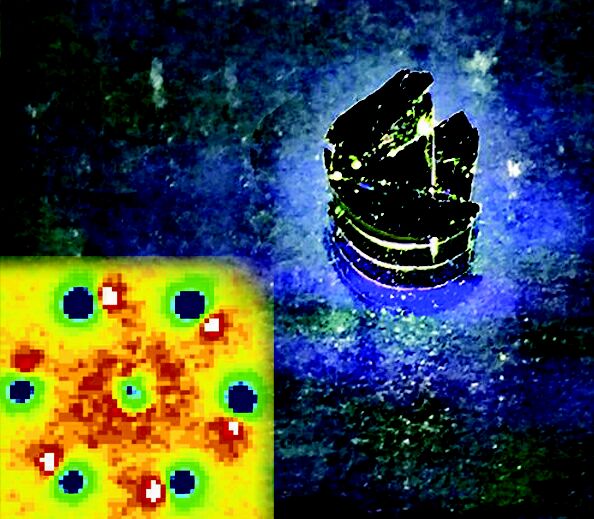 |
Resolution limit of ultrafast electron imaging and
spectroscopy
The UEM collaboration (groups of Berz, Duxbury, and Ruan) zoomed in on the stochastic space-charge effects in the generation of high-brightness electron beams. Using a multilevel fast multipole method, coupled with the shadow imaging of femtosecond photoelectron
pulses for validation, we quantitatively elucidate the photocathode, space charge and
virtual cathode physics, which fundamentally limit the spatiotemporal and spectroscopic resolution
and throughput of ultrafast electron microscope (UEM) systems[J. Appl. Phys. 111, 044316 (2012)]. A simple microscopic
description to capture the nonlinear beam dynamics based on a two-fluid picture and elucidate an
unexpected dominant role of image potential pinning in accelerating the emittance growth process.
These calculations set theoretical limits on the performance of UEM systems and provide useful
guides for photocathode design for high-brightness electron beam systems. electron imaging and spectroscopy [Appl. Phys.
Lett. 103, 253115 (2013)]. Further improvement in
time resolution may come through improving the source brightness, through for
example; laser pulse shaping or tuning of the driving photons to threshold energy
from a high eciency cathode; and may make ps macromolecular imaging
feasible without the need to outrun the radiation damage effects, as required in
the case of imaging using X-rays. Further quantitative details addresses the source-limited
performance of ultrafast electron imaging and spectroscopy systems under
various acceleration fields, and photo-electron pulse aspect ratios, namely
the so-called pancake and cigar scenarios. The simulation results point to the
interesting finding that a ps cigar beam indeed excels in coherence length ( up
to 20 nm with 10^4 electrons and ~1nm with 10^8 electrons, however the high
acceleration field also increases its energy spread, whereas the energy spread of
cigar shaped beams is quite unaffected[J. Appl. Phys. 116, 174302 (2014)].Overall, the fs pancake beam has significantly smaller longitudinal emittance up to the virtual cathode limit, and
is more suited for high combined temporal-spectral resolution UEDs, whereas
the cigar beam may be suited for ps imaging, close to the single-shot limit. These projections show promising parameter space for conducting ultrafast electron micordiffraction at close to the single-shot level,
which is supported by the latest experimental characterization of such a system [Proc. of SPIE, vol. 9198 (2014)].
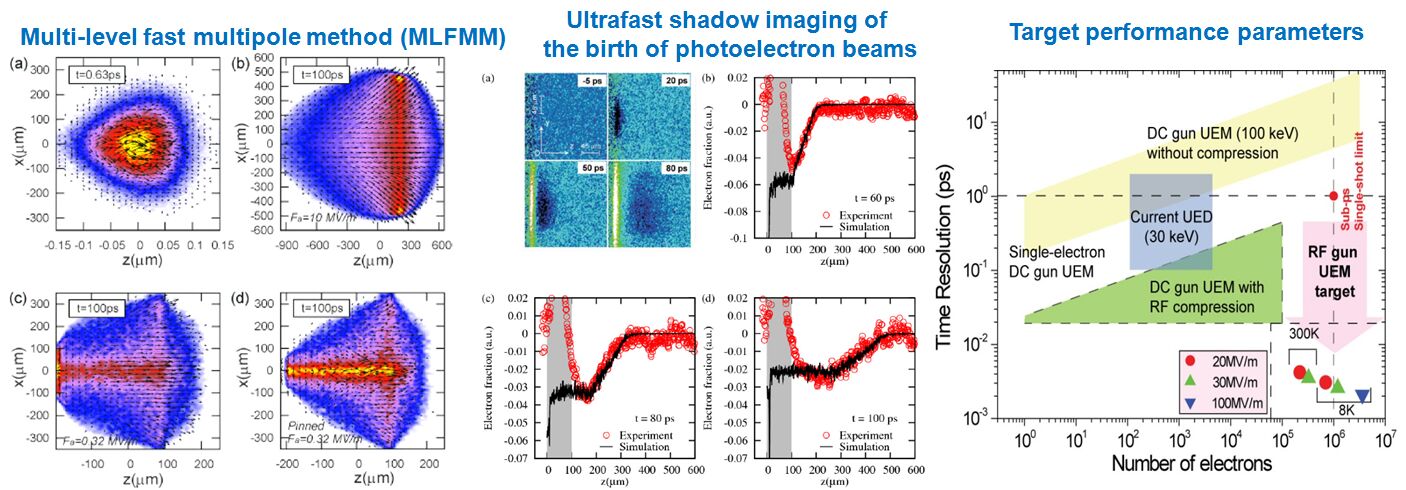
Decoupling of the electronic and structural phase transitions in VO2
Using
optical, TEM and ultrafast electron diffraction experiments we find that
single crystal VO2 microbeams gently placed on insulating
substrates or metal grids exhibit different behaviors, with structural and
metal-insulator transitions occurring at the same temperature for
insulating substrates, while for metal substrates a new monoclinic metal
phase lies between the insulating monoclinic phase and the metallic rutile
phase, which we show may be stabilized by charge doping. Informed by our
ultrafast single-beam measurements, the metal-insulator transition in the
undoped case can be classified as Mott-driven, Peierls-limited, where the
imposed cooperativity is mainly governed by the large spectral weight and
the high energy scale inherent to the Peierls transition and that such
stabilizing features are subjective to the strong Coulomb interaction
predominant only in the insulating state without doping. We also uncover
the unique delocalized nature of the interface-mediated charge doping
effect that can be rationalized with an active spectral weight transfer
from the unfilled band to the Peierls band in the monoclinic structure,
which enhances the Peierls interaction supported by its selective effect
on the structure phase transition temperature. While the results presented
here are very specific, they are generally compatible with the Peierls-Mott
scenario proposed by most recent theories, and amazingly reconcilable with
an array of different experiments supportive of either Peierls or Mott
scenarios. (Physical
Review Letter 109, 166406 (2012) ; Physical
Review B 87, 235124 (2013)) |
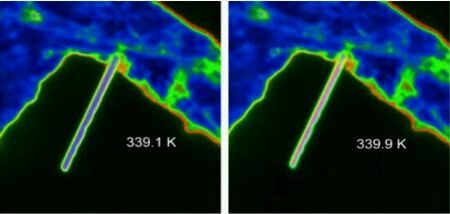 |
Tango dance of
electrons and ions in the phase transition of two-dimensional charge-density
waves
The coexistence of phases through complex couplings between different
degrees of freedom (spin, charge, lattice, orbital) is a hallmark of
complex materials with emerging properties such as charge density wave (CDW),
superconductivity, and colossal magnetoresistivity.
In particular, CDW is predicted to form in highly anisotropic
systems where long- range charge ordering (CO) and periodic lattice
distortion (PLD) are strongly coupled, driven by instabilities originated
in either the electronic (Mott)
or phononic (Peierls) subsystems.
Recent femtosecond spectroscopy-based pump-probe techniques offered
real-time characterizations of nonequilibrium dynamics in the different
regimes of electron-phonon coupling, but their properties are elucidated
in the temporal responses representative of mostly the evolution of CO
state. Using ultrafast
electron crystallography, we provided a complementary view from ultrafast
electron crystallography investigation of CeTe3, exploiting its
elegant 2D weakly correlated feature, serving as an ideal system to
understand the symmetry breaking phase transition in strongly coupled
electron-phonon system. We
specifically examined the fluctuational dynamics using the
momentum-dependent dynamical diffraction features to address the open
questions. Following a strong
perturbation of electron density, we observed clear evidences of
nonconcerted suppressions of CDW, expressed in the ionic frame as an
unexpected 2D lattice hardening and a short-lived topologically fragmented
state in the long-range PLD network resulted from CO suppression, and
highly nonuniform fluctuational amplitudes that are signatures of strong
anisotropic electron-phonon coupling driving PLD, respectively. The nature
of the transition was explained using a three-temperature model, in which
the first part of the dynamics can be reconciled with the optical
observations of electronic suppression; while the second part manifested
in the nonadiabatic ionic responses is highly system-dependent, and
informative of the electron-phonon mechanism for CDW formation.
(Physical Review B 86, 075145 (2012);
87, 235124
(2013)) |
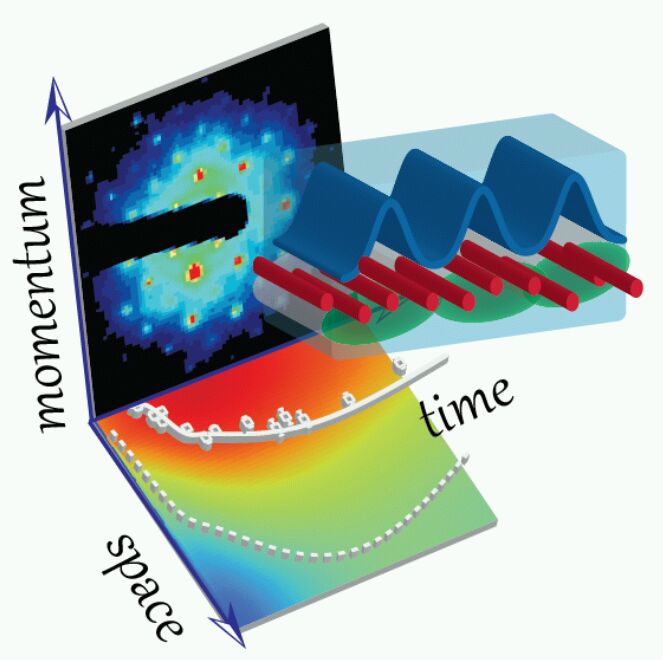 |
Imaging defects growth
in silver nanoparticles excited through surface plasmons
The ability to image defect growth processes is central to the understanding of the electronically induced structural phase transitions in solids. The recent emergence of using transient optical doping method to actuate atomic motions by modifying electronic structures of materials has opened up new vistas for exploring novel phases of materials. Whereas optical and photoemission studies have provided significant insights into the initial electronic processes that are strongly coupled to lattice degrees of freedom, the mechanism bridging the femtosecond (fs) optical seeding to the
ps-to-ns macroscopic structural changes remains a central topic to be elucidated. Recent developments in ultrafast diffraction techniques have enabled direct probing of atomic dynamics and helped accentuate the important role of electronic excitation in initiating the coherent motions, bond
softening and structural transformations. By employing ultrafast electron nanoscale crystallography
, we demonstrate a direct structural study of spatially inhomogeneous processes in Ag nanocrystals (NCs) induced via surface plasmon resonance (SPR) excitation. Contrary to an impulsive process leading to fragmentation, we find that the dominant dynamical feature in the prefragmentation stage is a defect-mediated instability growth, creating sub-nanocrystalline domains with hot surface and relatively cold core. Electronic effects are proposed to account for the incipient creation and subsequent growth of lattice inhomogeneities directly responsible for fragmentation, which are corroborated by the evidences of correlated charge localization and defect percolation on the picosecond timescale following
photoexcitation. (Physical
Review Letter 104, 123401 (2010) ) |
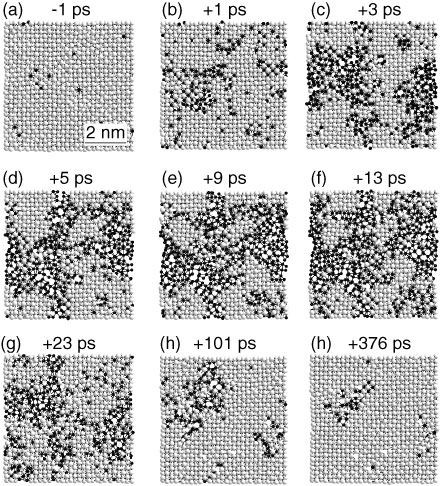 |
Imaging ultrafast
photoelectron dynamics
Understanding the mechanism of vacuum space charge emission and surface charge accumulation is crucial to the development of pulsed laser driven electron technologies, such as time-resolved photoemission, ultrafast electron diffraction and microscopy. Recently, a novel method to directly image the spatiotemporal evolution of the photoemitted electron packet generated over a femtosecond laser excited surface has been developed in
UEC group . This method possesses sufficient sensitivity to image electron density as small as
10^10 e/cm^3 and permits quantitative measurement of the instantaneous electron density distribution and its collected translational and expansion speed near the surface. These initial kinetic energy distributions are representative of the hot electron profile within the materials responsible for the photoemission. This technique thus can provide information equivalent to those obtained from time-resolved photoemission spectroscopy, but can be operated at a strongly driven condition with a direct resolution to distinguish the presence of space charge effects. In the study of photoemission from a graphite surface, we found that the dynamical profile of photoelectrons reveals an origin of a thermionic emission, followed by an adiabatic process leading to electron acceleration and reduction of electron expansion (cooling). The hot electron emission is found to couple with a surface charge dipole layer formation, with a sheet density several orders of magnitude higher than that of the vacuum emitted cloud. (Applied
Physics Letter 95, 181108(2009)) |
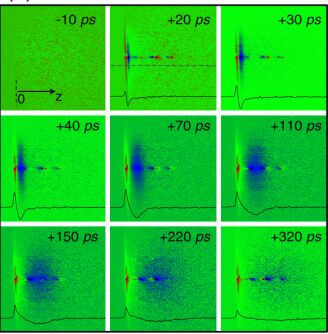 |
August 2009: MSU Strategic Partner Group formed to develop high-brightness
femtosecond electron microscope
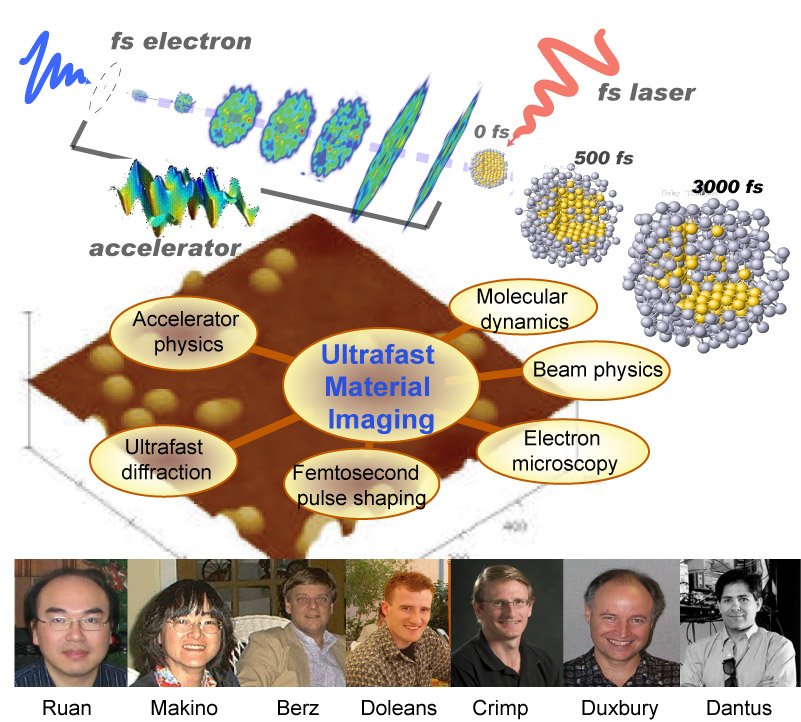
See PA announcement.
Quantitative modeling of excited state structural
dynamics to visualize surface melting of Au nanoparticles
Our group developed a progressive refinement method to
treat excited state ultrafast diffraction patterns by adopting a reverse Monte Carlo approach
to refine the excited state
structures . The tracking of particle dynamics is constrained by using the inherent dynamical correlations between structural functions obtained from two neighboring time frames. The ability of 'making molecular movies' is tantalizingly close from analyzing the photoinduced structural dynamics for the strong scattering
Au and Ag nanoparticles, based on this method.
(Microscopy and Microanalysis
Vol. 15, 323-337; Special issue on ultrafast electron microscopy and
ultrafast sciences) |
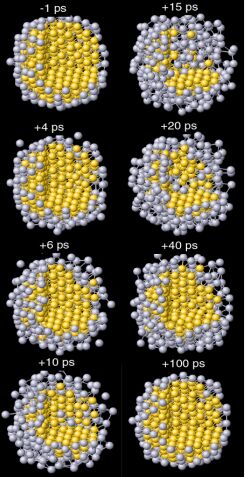 |
Shaping up graphite
with light
Can we make diamond from graphite without resorting to extreme heat and
pressure as diamond was made in the Earth in geological time? The UEC team raise such a possibility by
shining a short burst of intense femtosecond laser pulse on graphite and watching using ultrafast diffraction the movements of some loosely
separated carbon atoms in graphene layers undergoing rehybridization, forming more tightly bound diamond-like bonds. Whereas this intriguing
intermediate structure is short-lived, approximately for 30 picoseconds, this experiment shows the possibility of a structural transformation
purely induced by light in this very versatile class of material. Assisted by density functional theory
calculation performed by Tomanek's group at MSU, the cause for such a transformation is attributed to the electronic structure changes and the
Coulomb field buildup following the photoexcitation. We are currently
exploring different strategies in optically converting graphite into more
permanent form of diamonds on graphite surface and ultrathin slab.
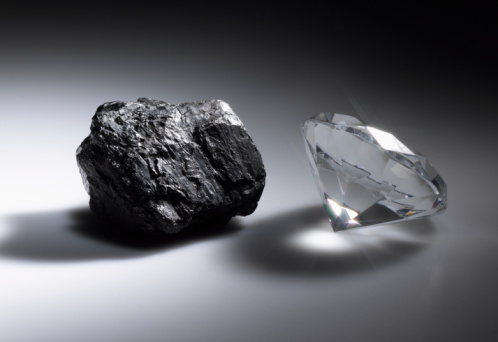
(PR focus article)
(Phys. Rev.
Lett. 101, 077401 (2008))
Measuring
photovoltage at ultrafast speed
We detailed a method to directly measure the photovoltage at
nanointerfaces using femtosecond electron pulses. The transient surface
voltage is determined by observing Coulomb refraction changes induced by
interfacial charging. Using coherent diffraction signal, we can trace the
origin of the refractive beam trajectory in material, thus obtain the nanoscale
scale sensitivity of the charge transfer process. We are currently
extending this approach to investigate molecular electronic processes.
(Phys. Rev. B 77,
245329 (2008)).
Ultrafast Meets Ultrasmall
Our
first paper outlining ultrafast electron nanocrystallography and the studies of
photomelting of size-selected Au nanoparticles is now published online (Nano
Letters: Author ASAP). Download PDF: with
subscription, without
subscription.
We report in the Nano Letters the studies of ultrafast electron
nanocrystallography on size-selected Au nanoparticles (2-20 nm) supported on a
molecular interface. Reversible surface melting, melting, and recrystallization
were investigated with full-profile atomic density maps determined with sub-picosecond
and picometer accuracies. In the ultrasmall environment, the melting process
induced by photons is ultrafast and far-from-equilibrium, transforming
nanocrystals into shelled nanoliquids. The
energy of the photons is transferred to the atoms within just a few picoseonds.
However, it takes tens of picoseond for the atoms to be released from the
crystalline motif into a liquid shelled structure. This dynamical transformation
is strongly influenced by a coherent shearing motion within fcc structure that
is determined as the pathway leading to the photomelting. The
nanoliquids is more icosahedral-like, and it reverts to the fcc crystalline state in
100 ps. The structural excitation
is displacive which results in a coherent transformation with crystal/liquid
coexistence and that differs from the reciprocal behavior of recrystallization,
where a hot lattice forms from liquid and then thermally contracts. The degree
of structural change and the thermodynamics of melting are found to depend on
the size of nanoparticle. The reversible and coherent transformation on the
ultrafast time scale demonstrates the directed dynamics on the energy landscape
of finite systems. This methodology is general and could be implemented to study
a wide class of phenomena pertaining to nanoscaled materials.
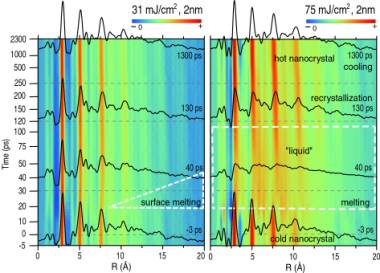
Time-resolved melting dynamics of 2nm Au nanoparticles. (Left)
Radial distribution function (RDF)
map constructed by stacking RDF of UEC patterns at a sequence of delays between
-5-2300 ps at irradiation fluence F=31 mJ/cm2. Surface melting
(enclosed by the dashed white line) is visible. (Right) RDF map for F=75
mJ/cm2. Full scale melting is observed. The liquid state (enclosed by
dashed white line) is characterized by the drop of 2nd nearest
density (at ~ 5Å) to (1-1/e) of the static value (at negative time).
|
|